
Mechanistic Services
Not sure if your reaction proceeds via radical or not? Reviewer 2 is not convinced by your proposed mechanism? We provide mechanistic studies for photo-activated reaction. Our toolkit includes: DFT investigation, rate constant measurements via Stern-Volmer quenching, quantum yield measurements and other spectroscopic methods.
Pictured: Proposed mechanism for...
Protein tagging

Above: In A), the labeling of a protein by photoredox mechanism from phenols and riboflavin from tetra-acetate. In B), biological applications of RFT-mediated photocatalysis in protein and cellular environments.
Protein identification technologies are essential for understanding fundamental biological processes and discovering new therapeutic targets. Visible light photocatalysis is an attractive platform for selective chemical transformations in biological materials with spatiotemporal control. The light excites a photocatalyst which activates chemical “tags” for the covalent labeling of proteins. Recently, we used riboflavin tetraacetate (RFT), a derivative of riboflavin, as a photocatalyst to label tyrosine-based proteins under blue light activation, especially in living cells and cell-cell contact areas. .
Photoredox catalysis
Photoredox catalysis is an emerging field of applied photochemistry, made possible by bright and efficient visible light sources. We explore the catalytic mechanisms of photochemical reductions by using computational chemistry to calculate various energies, allowing us to determine deactivation rate constants. All this contributes to the understanding of the kinetics and electron transfers within these photoredox catalytic cycles.
On the right is the phosphorescence of an iridium complex is completely attenuated upon addition of triethylamine; the same electron transfer mechanism can be used in photoredox to generate highly reactive radicals from simple LED lamps.
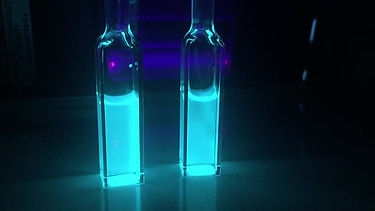
Reaction intermediates

Above: In A, the diagram of a flash photolysis system for a sample excited with an Nd:YAG source (1064 nm) doubled at 532 nm and measured using a spectrometer. The stopped-flow system also makes it possible to measure the absorption of a thermal mixture. In B, the same stopped-flow system used in freeze-quench mode and studied by Raman spectroscopy at low temperature.
Reaction intermediates are at the heart of all chemical mechanisms. Our laboratory tries to identify and characterize them. The use of fast spectroscopic methods such as flash photolysis (nanoseconds) makes it possible to detect photo-activated intermediates, but our efforts also focus on the stabilization of intermediates at low temperature in order to detect them by Raman spectroscopy. Using a stopped-flow device, liquid reagents can be mixed and then cryogenized to trap intermediates. A Raman analysis of this mixture at low temperature would reveal the fingerprint of this molecule - a method that would be much richer in information than absorption-based methods such as clear photolysis.
Spectroscopic and computational study of organic compounds and nanomaterials
Vibrational spectra are often considered unique "fingerprints" for each molecule, making them an accurate characterization method. We have developed software to perform quantitative comparisons between the Raman spectra predicted by theory and those measured experimentally. Moreover, we also use the prediction of Raman spectra to attribute the experimental peaks and to validate or not the molecular structure of the studied compounds.

Above: Image made with Blender representing ligands bound to gold nanoparticles with respective theoretical and experimental Raman spectra. The carbon-gold bond could be identified on the experimental spectrum thanks to the DFT prediction, thus confirming the covalent bond between the ligand and its gold nanoparticle.
Computational analyzes
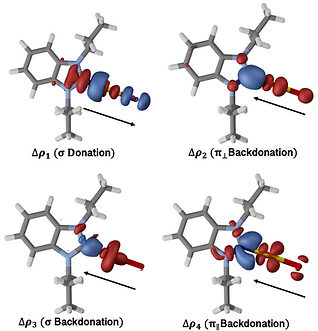
Our group has also used computational chemistry to demystify the various interactions present within a chemical bond. Through the use of the ADF program via the AMS software, we are able to decompose the binding energy and model these complex interactions by means of a simple calculation. The results obtained provide us with both a qualitative and quantitative understanding of the stability of all organic and inorganic compounds examined.
As mentioned in the previous page, we also use DFT to study the mechanisms of different systems (see pagemechanical service).
Left: Representation of strain densities (Natural Orbitals for Chemical Valence, NOCV) between fragments of a ligand and a gold atom. Red surfaces = loss of electron density; Blue surfaces = electron density gain.